My name is Charlotte, and I’m a computational neuroscientist – but what does that really mean?
It’s 5pm on a Friday afternoon. I’ve been in the office since 9am, staring at a bunch of equations and trying to figure out why they aren’t working like I expected them to. I’m still thinking about it on my walk home, as I unlock my door and toss my laptop onto my bed. It’s only once I’ve turned the kettle on that I remember I was meant to go buy milk. I’ve been running on autopilot.
Our bodies often have a mind of their own when we aren’t paying attention, and we only notice these unconscious actions when we plan to do something different. My research focuses on discovering how these subconscious actions are learnt, and, more importantly, how they may help us to understand and eventually treat addiction.
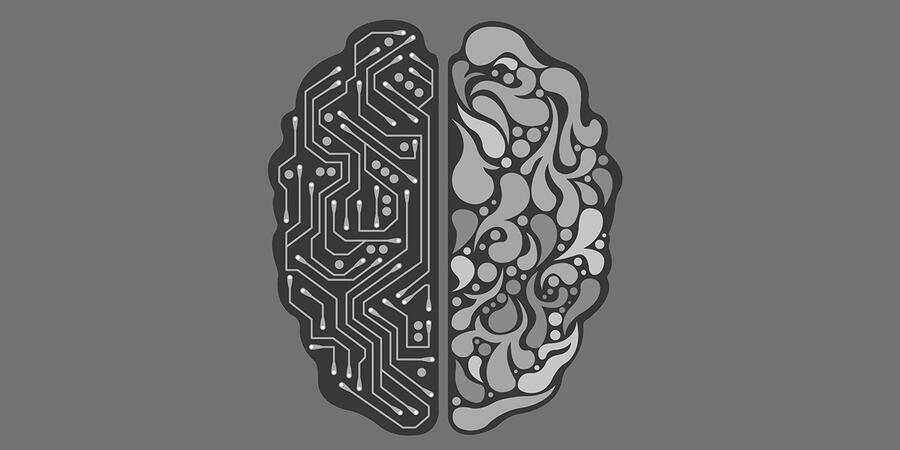
Brains are unimaginably impressive in all the processes they perform to keep us alive as conscious and complex humans, but, just like us, they sometimes cheat a little. Habits are one example of these shortcuts. When you see a certain stimulus often, and you always respond with the same action, your brain eventually stops caring about why you’ve picked that action and acts automatically – as a habit. I was leaving work, so I went straight home, forgetting about needing milk for my (crucial post-work) tea. Or I’m gazing into the abyss of my fridge for the eighth time today because I’m in my kitchen, having completely forgotten what I meant to do.
Normally, these habits are incredibly useful. They let us function more efficiently, and we don’t waste energy deciding how to do actions we’ve done a thousand times before. Instead, our brains focus on more important things, like solving work problems, or deciding what to have for dinner.
Unfortunately, habits can also go terribly, heart-wrenchingly wrong. The strongest versions of habits, called ‘compulsions’, are rare but almost completely unstoppable and are a key symptom in many brain diseases. These exist in addiction, OCD, and eating disorders. They also develop as a severe side-effect of Parkinson’s treatment. As scientists, we need to figure out exactly how brains create habits so that we can fight back when they go wrong. This is where my PhD comes in.
In the same way that I learn to check my fridge when I’m in the kitchen, my computer can also form habits. The simplest experiment I use is to give the computer a choice to (not) press a button. For three hours, every time the computer chooses to press the button it gets a reward, and a habit develops: “if a button is available, press it”. Then, I change the rules, and now every press causes punishment, not reward. If the computer wasn’t trapped in a habit, it would learn to stop pressing (which seems obvious from the outside). However, when my equations accurately mimic a compulsive brain, my computer should develop a compulsive habit instead and act automatically, no matter how much I punish it.
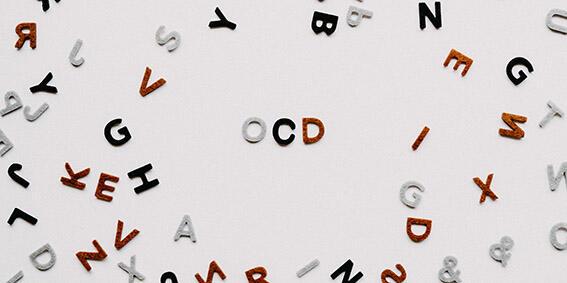
Unfortunately, habits can also go terribly, heart-wrenchingly wrong. The strongest versions of habits, called ‘compulsions’, are rare but almost completely unstoppable and are a key symptom in many brain diseases. These exist in addiction, OCD, and eating disorders. They also develop as a severe side-effect of Parkinson’s treatment. As scientists, we need to figure out exactly how brains create habits so that we can fight back when they go wrong. This is where my PhD comes in.
In the same way that I learn to check my fridge when I’m in the kitchen, my computer can also form habits. The simplest experiment I use is to give the computer a choice to (not) press a button. For three hours, every time the computer chooses to press the button it gets a reward, and a habit develops: “if a button is available, press it”. Then, I change the rules, and now every press causes punishment, not reward. If the computer wasn’t trapped in a habit, it would learn to stop pressing (which seems obvious from the outside). However, when my equations accurately mimic a compulsive brain, my computer should develop a compulsive habit instead and act automatically, no matter how much I punish it.
Experimental neuroscientists have already found two key receptors for action selection.
- Low dopamine levels activate the D2 receptor, which is found in the brain cells that tell us when acting is a bad idea. D2 activation makes these ‘No-Go’ neurons react more strongly the next time you see the signal you just responded to (e.g., the fridge). Essentially, D2 signals teach you to not repeat the action.
- In parallel, within the same brain region, high dopamine activates D1-expressing ‘Go’ neurons. These communicate that responding to the cue had a good outcome (lots of dopamine). Every action you do (or don’t) perform is decided through battles between these two neuron types, and my mathematical model describes how these fights play out.
This still isn’t a habit though, because it’s using the reward. So, my model investigates whether we can make the same dopamine system react to expected versus unexpected actions, instead of surprising rewards – and we have some interesting results already!
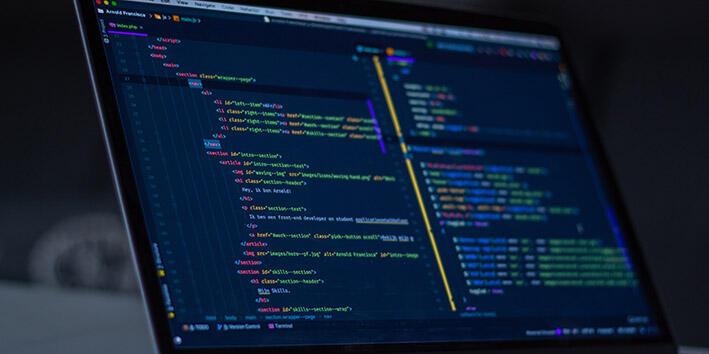
Looking ahead, once I prove that my model explains normal behaviour, I can then ‘break’ it – I can run simulations (no animals or humans involved) to see what happens when different parts of the brain system go wrong. Not only can this help us predict how this can produce disease symptoms, but it can also help guide future treatments!
I’m still in the first half of my PhD, but we’ve made great strides already. Hopefully, one day my ‘addicted’ computer will help treat my addiction TO my computer!
About the author
Charlotte Collingwood is a neuroscience PhD student who uses computers, maths and a biology background to learn more about how the brain works. She is currently doing a three-month internship with us, sharing her love for all things science. She can be found on Twitter at @NeuroCharlotte
Explore science at the Ri
Check out our weekly science talks, in-person and online, illuminating all areas of science—from physics to mathematics, engineering and neuroscience.